2022 Eilers Scholars
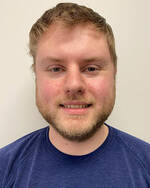
Michael Dugas
Understanding Electrostatic Interactions in Functionalized Copolymer Organic Solvent Nanofiltration Membranes
Department of Chemical and Biomolecular Engineering
Faculty Advisor: William Phillip
Organic solvent nanofiltration (OSN) has become an area of interest for organic solvent separation applications due to the energy efficiency, modular designs, and simple operations as compared to conventional thermally driven separations such as distillation. However, many of the polymeric membranes currently used in industrial applications cannot withstand the harsh chemical conditions. Copolymer membranes are able to be tailored using the physical properties of the repeat units in order to create membranes with distinct domains with distinct transport properties. In addition, functionalities can be incorporated into the membrane to not only crosslink and strengthen the nanostructure, but also incorporate charges into the membrane to electrostatically separate solutes. This type of interaction has been studied in a limited capacity in OSN.
This project is designed to investigate the effect of functionalities with known static charges on the transport of a poly(trifluoroethyl methacrylate-co-oligo(ethylene glycol) methyl ether methacrylate-co-glycidyl methacrylate) membrane that has been crosslinked with hexamethylene diamine. Investigating functionalities that exhibit a permanent positive charge, like a ternary ammonium, and a negative charge, such as a sulphonic acid, could help understand the outreach of surface and pore charge on the transport of ions in organic solvent environments. Analyzing the effect of the functionality on the dielectric constant of the membrane in each individual solvent, in addition to ion rejections, could also help to understand the rejections with known electrostatic models, such as Donnan Exclusion. Finally, by using the knowledge gathered in the fundamental studies, the membrane can be tailored to a specific application, such as removing a homogenous catalyst from organic solvents. Understanding the electrostatic interactions in organic solvents with the use of functional groups could help to further the applicability of OSN membranes and increase the energy efficiency of organic separation processes.
Project Objectives
Objective 1: Assess and characterize the functionalization of the copolymer membrane. The hypothesis is that by incorporating charged functionalities into the copolymer membrane, the electrostatic characteristics of the membrane will be altered. To assess this, copolymer membranes will be casted using both a flat-sheet blade casting and hollow fiber dip-coating methodology. The membranes will then be crosslinked with hexamethylene diamine using an epoxide-amine ring opening reaction. The charge groups will be incorporated after this reaction by either using an amine-isothiocyanate reaction, to get a sulphonic acid functionalization incorporated, or by utilizing another epoxide ring opening reaction, to incorporate a ternary ammonium group. Once the membranes are reacted, FTIR analysis will ensure that the reaction has taken place successfully. In addition, Zeta Potential as well as dielectric constant analyses will determine how the electrochemical potential of the membrane has changed with the incorporation of the functional group. This would help to understand the electrostatic characteristics of the membrane.
Objective 2: Understand how the functionalization effects the transport through the membrane. The hypothesis is with the incorporation of charged functionalities, the membrane will interact with solutes based on Donnan exclusion principles rather than pure size exclusion. To investigate this hypothesis, ion rejection experiments of sodium and calcium nitrate in varying organic solvents, such as methanol, ethanol, isopropanol, and hexane, will be conducted to determine the rejection of the salts in the organic environment. In addition, diafiltration experiments will be conducted to elucidate the effect of concentration on the rejection of the ions. These experiments, combined with characterization, can be utilized to link the rejection behavior to known electrostatic models in literature.
Objective 3: Utilize the knowledge and apply to industrially relevant feed solutions. The hypothesis is that by understanding the transport behavior of the membrane with each functionality, we can begin to accurately predict the transport behavior of industrially relevant feed streams through the membrane. One such example is to remove homogenous catalysts from the organic solvent to be recycled into the chemical process. In this study, homogenous charged catalysts will be separated from the products and organic solvents. This would demonstrate the ability of the membrane to separate solutes based on electrostatic interactions rather than pure size exclusion.
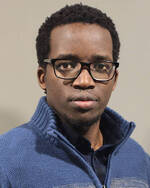
Anthony Kipkorir
Designing AgInS2-CdS Heterostructure with improved charge separations
Department of Chemistry and Biochemistry
Faculty Advisor: Prashant Kamat
Curious look into the future of energy demands points to the need utilize sustainable renewable energy to replace fossil fuels that have polluted the environment. Among many semiconductors, ternary quantum dots (QDs) particularly AgInS2 have gained popularity in recent years because of their potential applications in photovoltaic electricity generation and photocatalysis. Besides, such ternary QDs do not contain elements like lead that are harmful to environment. AgInS2 and its derivatives have bandgaps matching well to the solar spectrum. This feature along with composition-based band gap tunability and high absorption coefficients across the visible spectrum has given ternary quantum dots a niche in ongoing research.
The main drawback with this material is the existence of surface defects (trap states) which provide non-radiative recombination sites. These trap states originate from the non-bonding orbitals of the under-coordinated surface atoms. Use of ligands alone to stabilize the surface of QDs is not enough to address this problem. Recent research proposes modification of the surface AgInS2 QDs by coupling it with a higher band gap material to form a shell that eliminate the trap states (e.g., ZnS and CdS). Preliminary results from these studies shows that this surface modification significantly improve the QDs photophysical properties.
The band position of AgInS2 core and CdS shell allows for the design of quasi-type II heterostructure. This band alignment will allow the photogenerated charge carries to be separated thus allowing easy harvesting and utilization of the same. On the other hand, having a thick shell can hamper the rate of electron transfer as the electrons will have to tunnel through a longer distance. Therefore, understanding the role of shell thickness as a function of PLQY and electron transfer (ET) is crucial to optimizing the benefits that the shell bring. In this work, I will investigate how photoluminescence quantum yield (PLQY) and electron transfer (ET) process are affected with increasing shell thickness.
Project Objectives
Objective 1: The proposed study will begin with designing and synthesizing AgInS2-CdS heterostructure with varying CdS shell thickness. The presence of CdS shell and shell thickness will be probed using high resolution transmission electron microscopy (HR-TEM) (in the Notre Dame Integrated Imaging Facility (NDIIF)).
Objective 2: Absolute PLQY of the synthesized heterostructures will be determined using the integrated system available in Kamat laboratory. This information will help in tracking how the radiative recombination pathway is activated as the shell size is increased.
Objective 3: Excited state behavior of these material will be probed by employing ethyl viologen (EV2+) as a model electron acceptor. With the aid of photoluminescence spectroscopy, preliminary information on the interaction of the electron shuttler (EV2+) and AgInS2-CdS heterostructure will be obtained.
Objective 4: Consequently, insights on electron transfer processes will be obtained by using transient absorption spectroscopy technique (available in Kamat lab). The rates of electron transfer with varying shell thickness will be elucidated by monitoring how the EV2+ deactivates the excited states of the respective materials. These insights will be pivotal in designing efficient solar cell device or a photocatalyst.
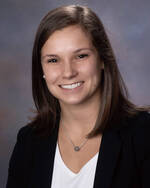
Deanna Poirier
Plasma-Assisted Catalysis for Upgrading Ethane to Valuable Liquid Products through Carbon-Nitrogen Coupling
Department of Chemical and Biomolecular Engineering
Faculty Advisor: Jason Hicks
Annually, over 140 billion m3 of valuable natural gas is released from wells during oil production [1]. Due to a lack of efficient storage methods, much of this natural gas is flared as a means of removal, releasing more than 350 metric tons of CO2 to the environment [1]. Since this flared gas contains light hydrocarbons, an alternative is to upgrade the gas to higher value products directly at the site of generation. Plasma-assisted catalysis is a relatively new technology that has promising results for this application. Plasma is an electrically generated source of reactive ions, radicals, and electrons. Applying these reactive plasma species to a catalytic reaction has shown improvements over traditional catalytic reactions [2]. Specifically, improved reaction kinetics at low temperatures and pressures have been observed, allowing for reduction of capital and operating costs [3]. Additionally, because renewable sources can provide the electricity needed to generate a plasma, on-site and mobile plasma units are a great opportunity for utilization of alternative energy. Natural gas upgrading with plasma has been shown to be possible, with one such reaction coupling methane, the main component of natural gas, with nitrogen. This reaction can produce nitrogen containing aromatic species, such as pyridine, which is a valuable liquid used in the pharmaceutical industry [4,5]. The challenge for this reaction is that it is not selective under plasma conditions, and often forms upwards of 10 different byproducts.
Catalysts such as ZSM-5 provide shape selectivity, meaning the size of the pores allow for the formation of products of a certain size. ZSM-5 is often used in the production of benzene, and therefore could assist in the formation of nitrogen containing aromatics when coupled with a plasma. Preliminary results show that using this catalyst and incorporating nitrogen into the hydrocarbon feed under plasma conditions leads to the production of pyridine, a chemical with a similar structure to benzene with the inclusion of a nitrogen in the ring. The combination of the plasma with the catalyst allows for pyridine production at temperatures of ~200 °C, which is lower than the current industrial temperature of 400 °C. Previous studies in the group have focused on the plasma-only reaction between ethane and nitrogen, investigating the impact of flow rate, feed composition, and power input in order to identify regimes that favor formation of nitrogen containing hydrocarbons. Ethane, the second largest component of natural gas, was chosen to study this reaction since it is easier to activate than methane; the knowledge gained will be helpful for later studies on methane, which is more difficult to couple with nitrogen. Guided by the knowledge gained from these previous non-catalytic experiments, this work will focus on incorporating a catalyst into the plasma reaction in order shift the selectivity towards pyridine. ZSM-5 is a tunable catalyst and can be synthesized with different acidities, as well as with different metals incorporated into the catalyst. Understanding how these factors affect the selectivity of the reaction will allow for improved performance of a reaction that can be used for on-site upgrading of hydrocarbons to valuable liquid products.
Project Objectives
Objective 1: Understanding the role of the acidity of ZSM-5 will be important for producing the desired products. High acidity generally leads to formation of aromatics, but rapid deactivation of the catalyst is often seen. Lower acidity tends to form less aromatics, but the catalyst often has a longer life. Understanding this trade off can help determine a suitable catalyst.
Objective 2: After understanding the effect of ZSM-5 on the reaction, various metals will be incorporated into the catalyst. The metal as well as the plasma will both activate the ethane, and the shape of the ZSM-5 will help form the aromatic compounds. This metal supported ZSM-5 could help to increase yields by converting more ethane to the aromatic precursors than the plasma alone, while the plasma continues to activate the nitrogen. Metals, such as platinum and platinum-tin, which are commonly used for ethane conversion to ethylene (an aromatic precursor) will be tested. Molybdenum and gallium, which are often used with ZSM-5 for methane aromatization, will also be investigated.
Objective 3: Previous studies in the group have shown that under plasma conditions, a simulated natural gas stream of methane, ethane, and propane combined with nitrogen forms similar products to the ethane-nitrogen reaction. After identifying a suitable catalyst for the ethane-nitrogen reaction, a simulated natural gas stream will be used to gain an understanding of how this reaction differs with a mixed stream. Being able to use a mixed feed eliminates having to purify the gas stream before upgrading.
[1] Calel, R.; Mahdavi, ,P. Proceedings of the National Academy of Sciences. 117 (23), 12503-12507 (2020).
[2] Mehta, P.; Barboun, P.; Herrera, F.; Kim, J.; Rumbach, P.; Go, D. B.; Hicks, J. C.; Schneider, W. F., Nature Catalysis. 1 (4), 269 (2018).
[3] Patil, B. S.; Wang, Q.; Hessel, V.; Lang, J., Catalysis Today. 256, 49-66 (2015).
[4] Bai, M.; Zhang, Z.; Bai, M.; Bai, X.; Gao, H. Journal of Air and Waste Management Association. 58 (12), 1616-1621 (2012).
[5] Ahmad, S.; Alam, O.; Naim, M. J.; Shaquiquzzaman, M.; Alam, M. M.; Iqbal, M. European Journal of Medicinal Chemistry. 157, 527-561 (2018).
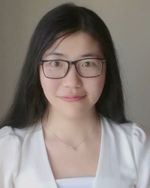
Hui Xu
Operando Surface Enhanced Raman Spectroscopy (SERS) Platform for Studying the Structure and Dynamics of Amine-based Membranes in Complex Environments
Department of Chemistry and Biochemistry
Faculty Advisor: Casey O'Brien
Separation and removal of CO2 from mixed gas streams are important in many industrial processes, including natural gas and hydrogen purification, as well as reducing greenhouse gas emissions. [1-2] Various strategies and technologies have been developed and used for CO2 capture in the past several decades, such as solvent adsorption [3-9], solid adsorption [3-8], and membrane-based separation.[3-8, 10] Among the various technologies, membrane separation, especially polymer-based facilitated transport membranes (FTMs) separation have been considered as a very promising technology with their inherent advantages, such as high energy efficiency, cost-effectiveness, operational simplicity, and flexibility to scale up. [6, 9-12] In these FTMs, the gas transport behavior is dominated by the facilitated transport mechanism, which exploits the preferential and reversible interaction between CO2 and reactive carriers to enhance CO2 permeability and selectivity. However, the limited understanding of the fundamental mechanisms of molecular- and nano-scale processes, which is crucial to membrane performance and changes over time especially in complex environments, has hindered the further development of the high-performance CO2 capture membranes.
To address the knowledge gap between polymeric membrane structure and performance, my work is to establish a new operando surface enhanced Raman spectroscopy (SERS) platform to monitor and detect the chemical structure and dynamics of membranes in realistic operating environments while simultaneously measuring the rate of CO2 transport across the membrane. Aspects of the CO2 transport mechanism can be elucidated by correlating the formation of intermediate species in the membrane to rates of CO2 transport across the membrane. We have successfully established such a new operando SERS platform and tested it with Polyvinylamine (PVAm) membrane, a commonly used amine-based membrane in CO2 separation due to the highest concentration of primary amino groups, as well as literature supported high CO2 permeance and selectivity. We show direct spectroscopic evidence of carbamate (NHCOO-) formation inside the PVAm membrane, which is the key intermediate for CO2 transportation across the PVAm membrane. As CO2 permeance of PVAm facilitated transport membrane is pressure-dependent, the effect of CO2 partial pressure on CO2 facilitated transport across PVAm membrane was also studied with our apparatus to study the trend and correlate the phenomenon with molecular- and nano-scale structure. We also demonstrate the utility of this new technique by investigating the mechanism of CO2 facilitated transport across different amine-type polymeric membranes (PVAm, PMVAm, PDVAm, and P4VP) during exposure to humidified CO2/CH4 gas mixtures. The new operando spectroscopy platform can also be applied to other types of membranes, such as mixed-matrix membranes, and ceramic membranes to provide detailed information under the realistic working circumstance, which could help to understand the key factors that determine membrane performance and design new membranes with enhanced performance.
Project Objectives
Objective 1: Design, develop, and test a new experimental operando Raman spectroscopic platform that could probe the chemical structure and dynamics of membranes in realistic operating environments. We aim to establish a new experimental operando Raman spectroscopic platform, and demonstrate the utility of the new operando SERS platform to characterize the molecular structure of Polyvinylamine (PVAm) membranes and simultaneously measure their gas separation performance under realistic operating conditions to detect and identify the intermediates that related to CO2 transport through the membrane.
Objective 2: Model study of Amine-Containing CO2-Selective Membrane Process for Carbon Capture. Based on our operando Raman spectroscopic platform, we want to correlate polymeric membrane structure and performance, and build a simple facilitated transport model for CO2 transport through PVAm (a type of amine-containing FTMs). The model could be used to roughly explain some experimental results and could also be used to modify membrane separation condition for different demands.
Objective 3: Use the new operando Raman spectroscopic platform to study and address the knowledge gap between different amine-based polymeric membrane structures (PVAm, PMVAm, PDVAm, and P4VP) and performance. We aim to investigate different amine-based membranes (PVAm, PMVAm, PDVAm, and P4VP) to understand the structure effect on CO2 transport mechanism, and to understand which amine types and operating conditions exhibit the optimal CO2 transport performance.
[1] Rufford, T. E.; Smart, S.; Watson, G. C. Y.; Graham, B. F.; Boxall, J.; Diniz da Costa, J. C.; May, E. F., The removal of CO2 and N2 from natural gas: A review of conventional and emerging process technologies. J. Pet. Sci. Eng. 2012, 94-95, 123-154.
[2] Yang, H.; Xu, Z.; Fan, M.; Gupta, R.; Slimane, R. B.; Bland, A. E.; Wright, I., Progress in carbon dioxide separation and capture: a review. Journal of environmental sciences (China) 2008, 20 (1), 14-27.
[3] Darabkhani, H. G.; Jurado, N.; Prpich, G.; Oakey, J. E.; Wagland, S. T.; Anthony, E. J., Design, process simulation and construction of a 100 kW pilot-scale CO2 membrane rig: Improving in situ CO2 capture using selective exhaust gas recirculation (S-EGR). J. Nat. Gas Sci. Eng. 2018, 50, 128-138.
[4] Lee, J.; Kim, J.; Kim, H.; Lee, K. S.; Won, W., A new modeling approach for a CO2 capture process based o a blended amine solvent. J. Nat. Gas Sci. Eng. 2019, 61, 206-214.
[5] Pellegrini, L. A.; De Guido, G.; Valentina, V., Energy and exergy analysis of acid gas removal processes in the LNG production chain. J. Nat. Gas Sci. Eng. 2019, 61, 303-319.
[6] Hemmati, A.; Rashidi, H.; Behradfar, K.; Kazemi, A., A comparative study of different mass transfer and liquid hold-up correlations in modeling CO2 absorption with MEA. J. Nat. Gas Sci. Eng. 2019, 62, 92-100.
[7] Hemmati, A.; Rashidi, H.; Hemmati, A.; Kazemi, A., Using rate based simulation, sensitivity analysis and response surface methodology for optimization of an industrial CO2 capture plant. Journal of Natural Gas Science and Engineering 2019, 62, 101-112.
[8] Wenten, I. G.; Khoiruddin, K.; Aryanti, P. T. P.; Victoria, A. V.; Tanukusuma, G., Membrane-based zero-sludge palm oil mill plant. Rev. Chem. Eng. 2020, 36 (2), 237-263.
[9] Babu, D. J.; He, G.; Hao, J.; Vahdat, M. T.; Schouwink, P. A.; Mensi, M.; Agrawal, K. V., Restricting Lattice Flexibility in Polycrystalline Metal–Organic Framework Membranes for Carbon Capture. Adv. Mater. 2019, 31 (28), 1900855.
[10] Míguez, J. L.; Porteiro, J.; Pérez-Orozco, R.; Patiño, D.; Rodríguez, S., Evolution of CO2 capture technology between 2007 and 2017 through the study of patent activity. Appl. Energy 2018, 211, 1282-1296.
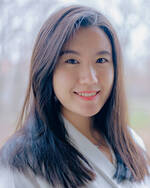
Lingyu Yang
Development of a Novel Polymer Membrane Platform for Alkaline Fuel Cell Electrolyte Application
Department of Chemical and Biomolecular Engineering
Faculty Advisor: Jennifer Schaefer
With the continually increasing demand for clean and renewable energy worldwide, tremendous efforts have been made to seek new energy alternatives. Electrochemical storage and conversion devices have been recognized as some of the most critical technologies in overcoming fossil fuel exhaustion and global pollution. Among these clean technologies, fuel cells outperform others because of high power generation efficiency, high specific energy, and low environmental pollution. Fuel cells represent environmentally friendly energy devices, including proton exchange membrane fuel cells (PEMFCs), and anion exchange membrane fuel cells (AEMFCs), et al. Ranging in potential application from grid-scale storage to the electrification of transportation, this research seeks to contribute to the pursuit of a future without a reliance on fossil fuels.
AEMFCs have many advantages over PEMFCs such as the wider selection of electrocatalysts, less metal corrosion, and lower cost. However, the anion exchange membranes (AEMs) reported previously were not as conductive and stable as the proton exchange membranes (PEMs), resulting in reduced performance in real applications. To address the issues of AEMs, the concept of novel AEMs was proposed which utilized a recently developed method, Friedel-Crafts hydroxylation. This project is in collaboration with Prof. Haifeng Gao’s group (Dept. of Chemistry and Biochemistry) and two different platforms (DFr and BrBB polymers)were designed. Only one other research group worldwide is pursuing this material platform. The group believes that this material platform has a high potential for success due to specific reasons (low-cost polymerization method, stability of backbone, et al) intrinsic to the platform. This work is concerned with using the above polymers as solid-state electrolyte to facilitate the movement of hydroxide, focusing specifically on how the polymer composition and structure impact species transport and electrochemical performances. Relevant measurements which have been conducted are the first use of fuel cell membranes in our research group and have established these measurement protocols.
Project Objectives
This work thus far has resulted in a systematical comparison between three model compounds whose structures mimic the prepared polymers, which identified polymer design requirements for effective long dangling modulation of quaternary ammoniums, leading to enhanced stability in alkaline media. Some potential good-performing structures have been screened. These encouraging preliminary data indicate this project has a high likelihood of yielding interesting and practical results.
To continue the research, the first objective is to characterize the relationships between polymer chain sequence (including both random copolymer and block copolymer), membrane mesoscale structure, ionic conductivity, and mechanical properties. It is the first time that the structure containing crosslinker is introduced into AEMs. Therefore, the structure-property relationship between varied crosslink density and QAs ratios will be first elucidated. In addition, here it is proposed that in BrBB polymer, crosslink density or hydrophobic ratio would dominate and change material morphology, which influences the mechanical performance of AEMs, and hence work is also attempted on fabricating BrBB polymer membrane with great mechanical robust structure, as well as outstanding alkaline stability via changing the above two parameters.