2023 Forgash Scholar
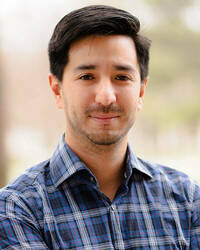
Raúl Torres-Cadena
Chemistry and Biochemistry
Faculty Advisor: Adam Jaffe
Hybrid Organic-inorganic Tungsten Bronzes as Tunable Optoelectronic Materials
Increasing worldwide energy consumption demands more efficient technology to produce, store, and utilize energy. In particular, the development of new and more efficient solar technologies can advance sustainable energy production and reduce the use of fossil fuels, thus securing a clean energy future. The performance of these systems relies on the structure and properties of new multifunctional materials. To be competitive, these new functional materials need to be less energy-intensive to produce, more cost-effective in comparison with similar materials, feature improved properties or easy tunability, and have a long lifetime.
Previously, tungsten oxide has been investigated for applications including solar cells and electrochromic devices (relevant for smart windows). Indeed, tungsten oxide exhibits intriguing properties relevant for integration in photovoltaic devices as either an electron transport layer or as a wide-band gap absorber in a multi-junction system. These characteristics include high electron mobility, a tunable band gap, moderate hole-diffusion lengths, and high transparency to light when existing in its oxidized state as WO3. Additionally, tungsten oxide has been explored for catalysis and energy storage, as well as for intriguing solid-state physical phenomena such as metal-insulator transitions and superconductivity. A primary reason for its detailed study is that WO3 can be reduced to form metal bronzes. A tungsten bronze is a non-stoichiometric compound with the formula AxWO3 (A+ = charge-balancing cation). The partial reduction of tungsten bronzes populates their conduction band, yielding free electrons with high carrier mobilities that enhance the chemical and electronic properties of the system, including by contributing to phase or metal-insulator transitions. However, the synthesis of inorganic tungsten bronzes can require high temperatures and lacks the chemical functionality of molecular systems. For this reason, I am working to develop hybrid organic-inorganic tungsten bronzes that contain two-dimensional metal oxides providing the favorable electronic and optical properties of the metal oxide, such as facile electron transport, strong optical absorption, and chemical stability. These 2D layers are then in proximity to organic molecules that can incorporate added functionality, such as catalytic or redox-activity, and especially photo-activity which is of particular relevance for solar technology. I am exploring synthetic control of these hybrid tungsten bronzes and studying their structure-property relationships. I will use a diverse range of diffractive, spectroscopic, and electrochemical characterization techniques to gain fundamental understanding of their optical and electronic behavior that will be relevant for solar energy conversion applications.
Research Objectives
1. Incorporating reduced 2D tungsten oxide layers in proximity to molecular organic arrays will lead to new functionality. We aim to develop synthetic control by judicious choice of organic interlayer species where we select for specific templating functional groups such as hydrogen bond donors and steric directing agents. We will also evaluate the role that reaction conditions such as pressure, solvent, pH, and temperature have in directing structure. Ultimately, we will build a library of related hybrid tungsten bronzes. This project will pay special attention to photoactive ligands that can enable more efficient charge separation or tune light absorption.
2. The electronic and optical tunability of these materials arising from their atomic arrangements will be studied using a variety of techniques, from structure characterization using diffraction experiments, to measuring the optical properties using spectroscopy, to analyzing the conductivity and the reduction potentials of these new materials using electrochemistry. The insights gained here will allow us to improve our synthetic efforts described in Objective 1.
3. Once tungsten oxide intercalates a cation to form the tungsten bronze, there is a shift in the electronic structure due to repositioning of the d-band, leading to a change in optical absorption and electronic conductivity behavior. Understanding of these phenomena are vital for implementation in solar technologies. I will pioneer computational investigation within my lab by performing density functional theory (DFT) calculations to better understand the complex properties of these new materials. To have more insight into the differences in electronic properties between the oxidized hybrid tungsten oxide and its reduced bronze form, I will analyze the band structure and the density of states of these materials and compare them with experimental results. Band structures will give me information about the band gap and its nature as either indirect or direct (which is relevant for light absorption), along with band dispersions that relate to charge carrier mobilities within these materials. Atom-specific density of states will give clues into how to chemically tune the electronic structure.
4. High-pressure experiments are a unique and powerful tool to gain fundamental understanding of the structural, electronic, and optical interplay within solids. High-pressure experiments will allow me to monitor structural changes and correlate them to optical and electronic properties that are important for the development of this material platform such as semiconductor-to-metal transitions, pressure-induced modulation of light emission, or charge transfer between organic and inorganic components. I have carried out preliminary studies of some of these new tungsten bronzes under high-pressure showing a possible pressure-induced metallization. Moving forward, I will explore the effects of compression on each new hybrid bronze and continue drawing connections between structure and properties.
5. Objectives 1–4 will lead to a greater understanding of structure-property relationships in our new hybrid tungsten oxide platform that will ultimately allow us to design these materials as tunable electron transport layers or semiconductor absorber layers for solar energy conversion. Molecular functionality can enhance our ability to integrate these hybrid materials within multicomponent devices, i.e., anchoring the hybrid to the next material layer in a device. Furthermore, incorporating photoactive ligands can enable light capture in multiple spectral regions or even promote more efficient electron-hole separation by transferring these photogenerated charge carriers to spatially isolated layers. Finally, the structure-directing behavior of the organic ligand can modulate the structure of the inorganic layer to tune the band gap. We will measure light absorption as a function of these effects and begin demonstrating proof-of-concept photovoltaic behavior.