2021 Eilers Scholars
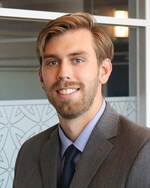
John Hoffman
Spatially-Controlled Functionalization of Nanofiltration Membranes
Department of Chemical and Biomolecular Engineering
Faculty Advisor: William Phillip
Membrane separations provide the most energy efficient means for producing clean water. As of 2017, an estimated 90 million m3 of water was produced via desalination daily. Of this capacity, 70% is produced by thermal processes through distillation, which requires large amounts of energy. However, because it is approaching the thermodynamic limit on separation efficiency,desalination by reverse osmosis (RO) membranes is rapidly displacing thermal methods. Despite the efficiency of RO, it remains energy intensive, which drives continued interest in wastewater reuse. In this regard, nanofiltration membranes (NF), which possess pores with diameters on the order of 1-8 nm have been developed. However, this porous nature results in a permeability/selectivity tradeoff, whereby balancing the performance of NF membranes to maximize selectivity with the highest possible permeability is needed. The functionality lining the pore walls of NF membranes offers a means through which to increase selectivity without sacrificing permeability.
A rapid and controllable reaction process that operates on time-scales relevant to modern roll-to-roll manufacturing is needed to functionalize the pore walls of NF membranes. “Click” reaction mechanisms are well-suited to address this need for precise, high throughput membrane functionalization techniques. This class of reactions is characterized by rapid kinetics as well as high conversions and selective yields. As such, click reaction mechanisms enable a vast number of functionalities to be incorporated with high fidelity on time scales consistent with membrane manufacturing rates. Copper (I)-catalyzed azide-alkyne cycloaddition (CuAAC) reactions are the premier example of a “click” reaction. Previous work within our group has shown the utility of this reaction for controlling the functionalization of nanofiltration membranes. Controlling the distribution of reactive sites across the membrane surface through inkjet printing applications result in domains that are larger than desired due to the physical limitations of the deposition process (i.e. droplet size, printer spacing, etc.). In order to better pattern the membrane surface, thiol-ene reactions, which are UV initiated, can be controlled and patterned through the use of photomasks. This offers an appealing opportunity to reduce the length scale of these patterns to better match the domain space in which interactions between the membrane and solutes exist. This work seeks to further develop the potential of thiol-ene based post-fabrication functionalization processes by developing a framework for understanding the factors that affect the ability to execute this reaction in a controlled manner and quantifying the resulting effects on the performance of patterned membranes.
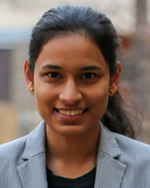
Preethi Susan Mathew
Understanding the Effect of Crystallinity on Perovskite Stability
Department of Chemistry and Biochemistry
Faculty Advisor: Prashant Kamat
Perovskite solar cells are coming up as the next generation solar cells with predicted cell efficiencies of up to 40 percent. They are much cheaper to produce than Silicon solar cells which are commercially used now. Perovskite materials allow for tuning of their bandgap, making it possible to absorb light of different wavelengths. This is possible by using Mixed Halide Perovskites (MHPs) which typically make use of Bromine and Iodine. However, these MHPs segregate under light irradiation. i.e., under light irradiation, a homogenous MHP separates into Iodide and Bromide rich domains. This significantly reduces the efficiency of a MHP solar cell.
Increased crystallinity of perovskite films has been proposed as a method to mitigate phase segregation. Phase segregation is promoted at grain boundaries in perovskite films, thus having increased crystallinity, or in other words increased grain size, should reduce phase segregation. This project will explore the effect of crystallinity of perovskite films on phase segregation. Specifically, it will look into how varying the grain size of perovskite thin films changes the extent of phase segregation under irradiation. It will also give a threshold grain size above which films will be stable from phase segregation.
Preethi Susan Mathew Final Report
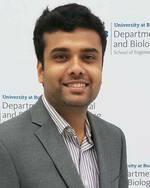
Krishnendu Mukherjee
Computational Materials Discovery and Design for Water Vapor Adsorption using Nanoporous Materials
Department of Chemical and Biomolecular Engineering
Faculty Advisor: Yamil Colón
Water is necessary for energy production due to its low-cost, high availability, and ease of use in the energy generation processes. It is also used as a medium for exchanging heat, for generating energy (via steam), for cleaning equipment, as well as for human consumption. However, water is not an unlimited resource and due to climate change, increase in energy demand, and rising global population, it is becoming scarce and unavailable to many parts of the world [1]. A solution to mitigate water scarcity is to devise novel technologies for recovering water vapor directly from air as well as from waste streams exiting from various industries. This extracted water vapor can be recycled back to the industrial and public facilities, and for this prospect, nanoporous materials such as metal-organic frameworks (MOFs) can offer a reliable, and tailored solution [2]. MOFs have the highest surface area per volume among all the crystalline materials, and many of them have demonstrated wide applicability in energy storage and separations including their use as atmospheric water harvester (AWH) materials [3,4]. My project aims to develop a computational method to study water vapor adsorption and separations in MOFs and determine how design variables such as pore size, geometry, electrostatics, and different functional groups affect the adsorption and separation mechanism. My Ph.D. project will leverage the established criteria to inform the rational design and discovery of porous materials for water vapor adsorption and separation.
Our preliminary results on water vapor interaction with idealized carbon cylinders (ICC) suggests that relative humidity (vapor pressure) has a significant role in affecting the adsorption phenomena. I found that the water vapor uptake in adsorption isotherm quickly rose to its highest water vapor uptake capacity beyond a pressure point, which signifies the presence of a limiting pressure after which the surface becomes hydrophilic. This study also confirms that charge arrangement on pore surface has a significant influence in influencing the hydrophilicity of ICCs. For example, the surface was found to be more hydrophilic when charges are alternated (from positive to negative) along the vertical axis (directed parallel to the pore length), then when they were alternated along the horizontal axis (perpendicular to the pore length). Electric potential maps and water vapor density isotherm for these cylinders further confirmed that charge arrangement plays a very important role in determining the hydrophilicity of these surfaces. We hypothesize that MOFs having similar electrostatic characteristics to the ICCs would also exhibit identical water vapor adsorption behavior and they can be potentially used for AWH technology and other water vapor separation processes. Further, novel MOFs may be designed by adding chemical moieties to existing MOFs to induce a desired water vapor adsorption and separation behavior as observed in the ICCs. In the next phase, I plan to develop an entropy-biasing Monte Carlo algorithm, since modeling of water vapor adsorption with MOFs has proven to be difficult due to large equilibration time and slow convergence rate. With the current MC algorithm, it is nearly impossible to simulate hypothetical structures from MOF databases such as inorganic crystal structure database (ICSD) and the Cambridge structural database (CSD). After developing this protocol, I wish to conduct a high-throughput screening to find the best performing MOFs for water vapor adsorption and separation applications.
References:
(1) Richey, A. S., Thomas, B. F., Lo, M.‐H., Reager, J. T., Famiglietti, J. S., Voss, K., Swenson, S., and Rodell, M.( 2015), Quantifying renewable groundwater stress with GRACE, Water Resour. Res., 51, 5217– 5238
(2) J. Canivet, A. Fateeva, Y. Guo, B. Coasne, D. Farrusseng, Water adsorption in MOFs: Fundamentals and applications. Chemical Society Reviews. 43 (2014), pp. 5594–5617
(3) Matthew W. Logan, Spencer Langevin & Zhiyong Xia, Reversible Atmospheric Water Harvesting Using Metal-Organic Frameworks. Scientific Reports. 10 (2020), Article number: 1492
(4) Kim, Hyunho, A Yang, Sungwoo, A Rao, Sameer R., A Narayanan, Shankar, A Kapustin, Eugene A, A Furukawa, Hiroyasu, A Umans, Ari S, A Yaghi, Omar M. A Wang, Evelyn N. Water harvesting from air with metal-organic frameworks powered by natural sunlight, Science, 430-434
Krishnendu Mukherjee Final Report

Agust Olafsson
Infrared Nano-spectroscopy of Plasmonic Materials Using High-resolution STEM-EELS
Department of Chemistry and Biochemistry
Faculty Advisor: Jon Camden
Understanding how to harness energy at the nanoscale is critically important for the rational design of the next generation of materials engineered to transduce charge, heat, or energy to their local environment. These properties are encoded in the local and bulk dielectric response as well as in particle morphology and cluster geometry, which give rise to the collective resonant excitations sustained by plasmonic and phononic materials [1]. Despite recent progress made using optical spectroscopies, a detailed picture of energy flow at the nanoscale is obscured by the diffraction limit, making spatially-resolved measurements increasingly difficult for low energy phenomena such as infrared (IR) plasmons and lattice vibrations. This research seeks to overcome this limitation by utilizing electron energy loss spectroscopy (EELS) performed in a scanning transmission electron microscope (STEM) to characterize the IR responses of plasmonic nanoparticles. We achieve this by using the current generation of aberration corrected and monochromated instruments which have significantly improved the ability to probe the IR regime with high energy resolution (<6 meV). STEM-EELS combines sub-nanometer resolving power with the capability to interact with a full spectral range of target excitations, making it the ideal technique to observe resonance behaviors for single particle and few particle systems [2-3]. These capabilities will be leveraged to detail the fundamental mechanisms of energy gathering and dissipation in plasmonic systems that underlie a broad variety of energy focused applications such as IR-photocatalysis, photovoltaics, surface-enhanced spectroscopy, and more [4-5].
[1] Maier, Stefan Alexander. Plasmonics: fundamentals and applications. Springer Science & Business Media. 2007.
[2] Masiello et al. Characterizing Localized Surface Plasmons using Electron Energy-Loss Spectroscopy. Annu. Rev. Phys. Chem. 2016, 67, 331-357.
[3] Camden et al. Probing nanoparticle plasmons with electron energy loss spectroscopy. Chem. Rev. 2017, 118(6), 1994-3031.
[4] Hutter et al. Exploitation of localized surface plasmon resonance. Advanced Materials, 2004, 16(19), 1685-1706.
[5] Willets et al. Localized surface plasmon resonance spectroscopy and sensing. Annu. Rev. Phys. Chem. 2007, 58, 267-297.

Yujia Wang
Computational Investigation of the Identity and Reactivity of Exchange Cu Sites in Zeolites for Selective Activation of Methane
Department of Chemistry and Biochemistry
Faculty Advisor: William Schneider
The increases in shale gas production in the world has driven the increase in global methane abundance. Methane is the main hydrocarbon in natural gas as well as a greenhouse gas. Converting methane to water and carbon dioxide through combustion can release energy. Converting methane to methanol, however, would form a liquid compound that can be transported more easily and subsequently converted into a variety of chemicals and fuels. Cu-exchanged zeolites, a type of heterogeneous catalysts with well-defined porous structures, have only recently been found to be active for partial methane oxidation to methanol (PMO), but the activities of the catalysts are still very low. Further advances require a fundamental molecular-level understanding of the structure and function of the active sites and the selective oxidation mechanisms in order to design catalysts that react with molecular oxygen to oxidize hydrocarbons. Cu-exchanged zeolites, on the other hand, are remarkable catalysts in terms of reactivity, selectivity and stability for NOx conversion to N2 (selective catalytic reduction of NOx with NH3, SCR). My previous research on dimeric Cu species in Cu-SSZ-13 zeolite for SCR shows Cu sites have a strong propensity to form oxygen-bridged dimers which would make O2 activation and CH4 activation possible for PMO reactions. Our experimentalists collaborators from Professor Gounder’s group at Purdue University are striving for the development of new tools and technologies for continuous methane oxidation to methanol on Cu-exchanged zeolites. Combining his group’s experiment effort with my molecular-level modeling and simulations, we would advance the fundamental mechanistic understanding of the reaction and the catalysts and eventually diversify the strategies available to use the world’s abundant hydrocarbon resources.
Systematic investigation of the stability and reactivity of exchange Cu sites at different locations of the zeolite (different framework Al proximity) and at various reaction conditions using density functional theory (DFT) will be conducted to identify the active Cu sites and to understand their oxidation-reduction behaviors. Monte Carlo simulations of global Al distributions will be conducted to bridge the knowledge of microscopic details of monomeric or dimeric Cu and reactivities of exchange Cu cations to the macroscopic composition of the zeolites (Si:Al and Cu:Al ratios) to predict the quantities of active Cu sites. This workflow can be applied broadly to other zeolites to predict speciation and distribution of exchange cations as consequences of Al distributions, and to find optimal zeolite compositions for catalysis applications.