2020 Eilers Scholars

Shelby Brantley
Understanding the Dynamics of Solid-Electrolyte Interphase in Sodium Ion Batteries with Fluoroethylene Additive
Department of Chemistry and Biochemistry
Faculty Advisor: Steven Corcelli
A primary limitation of rechargeable batteries is the build-up of the solid electrolyte interface (or interphase, SEI) on the electrode surface. The formation of the SEI causes irreversible capacity, cycle lifetime, and rate capacity restraints for sodium batteries. Additives, such as fluoroethylene (FEC), can reduce or even eliminate the side reactions that cause the SEI, however, they have been used sparingly due to the lack of fundamental understanding of the mechanisms that produce the SEI. In collaboration with the Krummel group at Colorado State University, my goal is to use a combination of molecular dynamic simulations and 2D IR data are used to gain a fundamental understanding of liquid electrolyte solutions.
Liquid electrolyte solutions of dimethyl carbonate, ethyl carbonate, ethylene carbonate, and fluoroethylene carbonate are experimentally studied by adding methyl thiocyanate as a probe and identical solutions are simulated using the polarizable force field, AMOEBA. Using correlation functions, the dynamics of the solutions can be evaluated on a molecular level using molecular dynamics and related to the 2D IR data for validation and interpretation. Electric field correlation functions provide information regarding the whole solution dynamics by calculating the electric field on the nitrogen of the probe and directly relate to the center line slope. Further evaluation using rotational correlation functions for each solvent molecule provides data indicating which solution component is contributing to the changing the solution dynamics measured by the probe. Using cylindrical distribution functions, the solvent shell can be visualized to determine which solution components are in the solvation shell of the probe. Combining all of the information, a better understanding is formed of which components are contributing to the changing dynamics of the electrolyte solution, and therefore, a more fundamental understanding of how the additive, FEC, changes the dynamics of the liquid electrolyte solution is developed. This can be further built on to understand what decomposition reactions are present and how the dynamics of the solution effect these mechanisms.
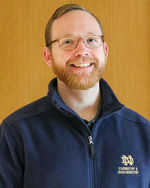
Jeffrey DuBose
Understanding How Phase Segregation Occurs in Mixed Halide Perovskite Solar Cells
Department of Chemistry and Biochemistry
Faculty Advisor: Prashant Kamat
Solar cells utilizing a perovskite as the light absorbing material are the next generation of high performing and lightweight solar panels. The state-of-the-art perovskites contain a mixture of different halide atoms in the crystal structure – typically bromine and iodine. In a solar cell utilizing these mixed halide perovskites (MHPs), the MHP is in contact with a charge extraction layer which pulls electrons out of the cell. This project will investigate how one of the most common electron transport layers – titanium dioxide (TiO2) –– can induce a phase change in MHPs under operating conditions. Specifically, it will investigate how electron transfer out of the perovskite can cause the MHP to segregate into regions that are more iodine-rich and bromine-rich, due to a build-up of holes (positive charges). Understanding the role of hole accumulation in phase segregation is crucial, as segregation drastically lowers the performance of a solar cell[1]. Developing a better understanding of phase segregation will allow scientists to develop strategies to mitigate the process and stabilize these materials.
[1]: Samu et al., ACS Energy Lett. 2017
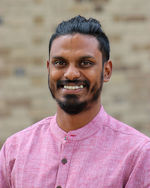
Elvis Eugene
Revolutionizing Lithium-Ion Battery Recycling with Membrane Separations: Multiscale Modeling, Optimization, and Uncertainty Quantification
Department of Chemical and Biomolecular Engineering
Faculty Advisor: Alexander Dowling
The importance of lithium-ion batteries (LIBs) is evident from their ubiquity in society and the 2019 Nobel Prize in Chemistry. Despite great advances since the 1970s, there remains a pressing need for sustainable LIB recycling technologies. Furthermore, worldwide production of lithium (Li), cobalt (Co), and other raw materials are insufficient to meet their projected demand over the next decade1 necessitating their secondary mining (recycling) from spent LIBs. Traditional processes that recycle LIBs are energy intensive and use large volumes of environmentally insulting solvents and high strength acids in leaching processes to extract metal rich solutions for further processing2. Relative to conventional technologies, membrane separations have demonstrated significant advantages in sustainability and energy efficiency3. Specifically, self-assembled copolymers, due to their nanoporous structure and pore wall functionalization enable the separation of similarly sized solutes via chemical affinity and can replace the solvent used in current LIB recycling technology. However, there is no clear understanding of the interfacial and thermodynamic phenomena underlying the chemically-selective transport mechanisms needed to separate molecules with comparable sizes such as Li and Co (atomic radii 1.8 Å and 2.0 Å respectively) which hinders the development of membrane materials for this task. Another challenge is the recovery of high purity products from a membrane separations unit. The formation of salt precipitate layers at the membrane interface when the feed side solution approaches the solubility limit prevents the extraction of high purity products, which also prevents the direct staging of these units.
References: 1. Bloomberg NEF Electric Vehicle Outlook, 2019; 2. X. Zeng et. al., Crit. Rev. Environ. Sci. Technol., 2014, 44, 1129; 3. W. A. Phillip, et al. Science, 2011, 333, 712;

Hunter Ford
Electrochemically Stable Ionomers with Minimal Sulfur Affinity for Mitigating the Polysulfide Shuttle in Metal-sulfur Batteries
Department of Chemical and Biomolecular Engineering
Faculty Advisor: Jennifer Schaefer
The mitigation of fossil fuel driven climate change is a major global challenge, one that can be addressed only by a large-scale shift towards sustainable energy. For this large-scale transition to be realized, the intermittency associated with solar and wind based energy generation must be managed. A logical approach to overcome this barrier is to store the energy produced when the sun is shining and wind is blowing so the energy can be used later when needed. My Ph.D. research is concerned with developing better energy storage technologies that are designed to meet the energy needs of the future. Ranging in potential application from grid-scale storage to the electrification of transportation, my research seeks to contribute to the pursuit of a future without a reliance on fossil fuels. The standard lithium-ion battery delivers insufficient storage capacity for next generation technologies like long-range electric vehicles. Furthermore, the electrode materials consist of transition metals such as cobalt, the mining of which poses health hazards and raises numerous ethical concerns.1 New battery chemistries that address these shortcomings are highly desirable. Metal-sulfur batteries are potentially one such set of chemistries, coupling an alkali or alkaline earth metal anode with a sulfur based cathode. Metal-sulfur batteries can have theoretical energy capacities at least six times higher than commercially available lithium-ion, and can address both grid-scale and transportation storage needs.2 The versatility of the metal-sulfur chemistry, as well as the widespread abundance of sulfur and relevant metals such as magnesium, makes this an attractive and sustainable technology. Metal-sulfur batteries are not without their own set of unique challenges. Intermediate species called polysulfides are formed during the battery operation. These species are necessary for the storage and release of energy. The challenge is that polysulfides dissolve in the liquid electrolyte of the battery, where they can diffuse out of the cathode and reach the anode. Polysulfides can passivate the surface of the metal anode, and/or get trapped in a process of constant redox shuttling between the electrodes, both of which result in short lifetimes of metal-sulfur batteries and inefficient energy usage.3 Addressing this phenomenon, known as the polysulfide shuttle, is paramount for enabling the practical use of metal-sulfur batteries and is the subject of my Ph.D. research.
My research has previously shown that certain ionomers (polymers that contain covalently tethered charges) are effective at mitigating the polysulfide shuttle in metal-sulfur batteries. When employed as a separator, a component that is sandwiched between the cathode and anode in a cell, I have demonstrated that certain ionomer chemistries can successfully diminish the polysulfide shuttle in magnesium sulfur batteries. Although the performance of the cells with ionomer separators was improved, unfortunately there was still a large degree of capacity fading.4 The conclusions of that study as well as preliminary unpublished results have identified that the capacity fading was due in part to the absorption of the sulfur into the ionomer. The ionomer successfully prevented the sulfur from contacting the magnesium anode, thereby interrupting the polysulfide shuttle, but because the sulfur was absorbed into the ionomer the cell still suffered capacity fading. I have recently identified a series of ionomer chemistries that have low sulfur affinity, meaning that these materials should not absorb the active material and therefore lead to higher capacity retention. However, while these materials do not absorb sulfur under passive diffusion conditions, they appear to react with sulfur when in a full metal-sulfur cell. This electrochemical instability seems to result in the sulfur becoming covalently bound to the ionomer, once again resulting in a loss of capacity upon cycling. The identified materials that passively reject sulfur will be modified to no longer contain the electrochemically unstable components, resulting in an ionomer that will not absorb nor react with sulfur. In developing an electrochemically stable ionomer with low sulfur affinity, the performance of metal-sulfur batteries is expected to be dramatically improved.
(1) Banza Lubaba Nkulu, C.; et al. Sustainability of Artisanal Mining of Cobalt in DR Congo. Nat. Sustain. 2018.
(2) Manthiram, A.; et al. Rechargeable Lithium-Sulfur Batteries. Chemical Reviews. 2014, pp 11751–11787.
(3) Kim, H. S.; et al. Structure and Compatibility of a Magnesium Electrolyte with a Sulphur Cathode. Nat. Commun. 2011, 2 (1).
(4) Ford, H. O.; et al Cross-Linked Ionomer Gel Separators for Polysulfide Shuttle Mitigation in Magnesium-Sulfur Batteries: Elucidation of Structure-Property
Relationships. Macromolecules, Front Cover Featured (2018), 51 (21), 8629-8636.
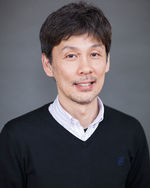
Mitsugu Hasegawa
Development of a Drag Reduction Technique Using a Microfiber Coating Inspired by Hair-follicles on the Seal
Department of Aerospace and Mechanical Engineering
Faculty Advisor: Hirotaka Sakaue
Transportation systems including cars, trains, and aircraft consume extra fossil fuel due to aerodynamic drag. Technologies that reduce drag are useful in improving fuel efficiency, which in turn benefits both the environment and the economy. Coatings are a key technology because of their broad applications, including their use in modifying existing technologies. One such coating is a novel bio-inspired hairy surface designed to reduce drag. It is known that many aquatic mammals, such as seals have a thin coating of hair. In addition to providing insulation and protection to aquatic mammals, it is theorized that this hair allows them to smoothly move through water. With this in mind, I sought to develop a coating to mimic the hair follicles on seals in order to reduce drag. The project has been sponsored by The Air Force Research Laboratory (AFRL) and Tanaka-Ai for the development of drag reduction techniques using surface and coatings. I have developed different coatings of various thicknesses with imitation hair follicles using nylon fibers. I have been conducting wind tunnel campaigns using different hair coatings on both a cylinder and airfoil test model. The information gained through performing these tests will be used for the demonstration, characterization and theoretical foundation of drag reduction using a hairy surface.