2017 Eilers Scholars
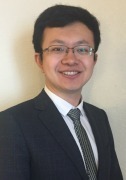
Hanyu Ma
Size Effect on Ruthenium Nanoparticle-Catalyzed Hydrogen Generation from Hydrazine Borane
Department of Civil and Environmental Engineering and Earth Sciences
Faculty Advisor: Peter Burns and Chongzheng Na
Hydrazine borane (N2H4BH3) is a promising hydrogen storage material because of its high hydrogen content (15.4 wt %), high stability under ambient conditions, and nontoxicity. Hydrogen stored in N2H4BH3 can be released by either catalytic solvolysis or thermal decomposition reactions. Thermal decomposition is often considered preferable because solid N2H4BH3 instead of saturated N2H4BH3 solution is used to maximize the storage capacity. However, thermal decomposition requires high temperature for efficient H2 release and it often generates significant amount of byproducts. In comparison, high purity hydrogen can be released from N2H4BH3 through metalcatalyzed hydrolysis under ambient conditions:3 N2H4BH3 + 2 H2O == N2H5+ + BO2- + 3 H2. Given the urgency of replacing fossil fuels with clean and renewable energy sources such as hydrogen, it is not surprising that a wide variety of nanocatalysts and their alloys have been investigated to improve the efficiency of N2H4BH3 hydrolysis. Surprisingly, however, the size effect of the nanocatalyst has been overlooked and remains unknown. In our previous study, size has been found to be a crucial design principle of the catalyst for hydrogen generation from hydrolysis of ammonia borane (NH3BH3 + 2 H2O == NH4+ + BO2- + 3 H2). The size effect induces compensation effect and produces an isokinetic temperature (Ti) at 17.5(±1.6) °C, at which ruthenium nanoparticles with different sizes show the same zero-order rate constant. The rate constant of the reaction can be maximized for operations performed below Ti by reducing the size of nanoparticles, which increases the fraction of edge and corner atoms and lowers the activation energy. To generate hydrogen above Ti, rate constant is maximized by using enlarged Ru nanoparticles with higher activation energies.

Randal Marks
Selective conversion of nitrate to ammonia using earth-abundant hydrogenation catalysts
Department of Civil and Environmental Engineering and Earth Sciences
Faculty Advisor: Kyle Doudrick
The Haber Bosch process, which converts N2 to ammonia, is at the heart of the food-energy-water nexus, providing fertilizer to produce food necessary for sustaining life. However, as this process is predicated upon nitrogen fixation—breaking the extremely stable N≡N bond—it is extremely energy intensive (consuming up to 2% of global energy) and has unbalanced the natural nitrogen cycle, resulting in contamination of surface and ground waters with waste nitrate. Bypassing nitrogen fixation by converting nitrate to ammonia offers a transformative approach to low energy ammonia production, while improving each component of the food-energy-water nexus. The objective of this project is to develop a transformative low-energy ammonia formation technology by engineering a nanostructured hydrogenation catalyst that uses earth-abundant elements for selective conversion of aqueous nitrate to ammonia. This project comprises of multiple distinct phases. The initial literature review is largely completed and candidate metallic catalysts have been selected. Iron, zinc, copper, nickel, and molybdenum disulfide will be explored for their ability to selectively convert nitrate to ammonia under hydrogenation conditions and their relatively high earth abundance. The activity and selectivity of these catalysts for nitrate reduction in conjunction with reducing agents H2, sodium borohydride, and formic acid will be determined by batch experimentation. Determination of reaction parameters, including nitrate sorption mechanisms, reaction kinetics, and intermediate/byproduct formation will be analyzed using time resolved infrared spectroscopy-attenuated total reflectance- Fourier transform infrared spectroscopy (TRISATR-FTIR). Thermodynamic parameters such as entropy and heat of adsorption will be analyzed using isothermal titration calorimetry (ITC).
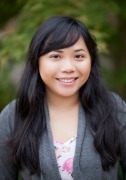
Triet Nguyen-Beck
Rationalizing the Size-dependent Stoke Shifts in Cesium Lead Bromide Perovskite Nanocrystals
Department of Chemistry and Biochemistry
Faculty Advisor: John Parkhill
The Kuno group has observed a sizable (up to 100 meV) growth in Stokes shift in small (<15 nm) nanoparticles (NPs) of cesium lead bromide perovskites. We will investigate and explore possible explanations for this phenomenon by performing electronic structure calculations.
Triet Nguyen-Beck Final Report

Jize Zhang
A Comprehensive Computational Framework for Layout Optimization of Wave Energy Converters that Addresses Large Dimensional Arrays, Causal Control and Uncertain Wave Environments
Department of Aerospace and Mechanical Engineering
Faculty Advisor: Alexandros Taflanidis
In the transition of the US energy economy from traditional technologies to renewables, ocean wave energy technology has a significant role to play. Almost all of the wave energy technologies involve the employment of Wave Energy Converters (WECs) -- some dynamically excited vibrating structures which interact with periodic ocean swells/waves. By interfacing this induced motion with generators, wave energy can be harvested, conditioned, and delivered to a power grid. For WEC technologies to be relevant on a utility scale, it will be necessary to distribute vast arrays of energy-harvesting buoys across a wide expanse of ocean, as scaling up individual WECs to the several megawatts is economically impractical. Unlike wind farms, which are typically arranged to minimize interference between the wind turbines, certain layout of WECs in an array can induce constructive hydrodynamic interactions between WECs, i.e., such interaction increases the power generation. Such effect gives rise to the layout optimization issue. However, the hydrodynamic analysis and performance estimation of different layout for WEC arrays have to be carried out using high-fidelity numerical models and thus becomes computationally expensive. Computational demand increases rapidly with the array size, making the determination of optimal layouts of WECs in arrays extremely challenging, even for arrays as small as 3-4 WECs. As such, the present state-of-understanding for layout optimization is still limited to small array sizes. Furthermore, to simplify the challenging optimization problem, most existing approaches ignore the fact that sea states are random and uncertain phenomena, and assume anti-causal controllers for WEC, thus requiring some anticipatory technique (i.e., present decisions for the WEC controllers are made assuming that future wave status is exactly known) which is frequently impractical to utilize in real-world applications. As a consequence, they often result in sub-optimal performance, especially in the presence of uncertain sea states. The proposed research constitutes an effort to rectify these issues and deliver an accurate and efficient approach for assessing and, more importantly, optimizing the power generation potential of WECs addressing large scale arrays, causal control implementation and consideration of random characteristics for the sea state. The ultimate goal is the following: for a given area and a given geographical site (defining wave environment) find the optimal number and optimal configuration of a WEC array so that the power extracted from the sea is maximized. The computational demand associated with this optimization will be reduced by the incorporation of a statistical emulator (meta-model) that can efficiently predict the hydrodynamic interactions based on small number of evaluations of the initial, computationally expensive numerical model. Uncertainties both associated with such emulator prediction and with the wave environment will be characterized and incorporated into the formulation of the associated layout optimization problem. Modern control theory will be further integrated by employing a causal controller (i.e., do not explicitly require future waves to be known), which makes power generation decisions based only on easy-to-measure feedback signals such as the generator velocities or their voltage. The result will be that for a given set of assumptions regarding the probabilistic uncertainty associated with the sea state, the resultant WEC formation to emerge from this approach will be truly optimal; i.e., no other formation, regardless of how it is controlled, will out-perform it. Once developed, the technique can be applied for both for site scoping as well as actual array design.