2023 Slatt Scholars
Reinhard Bartsch
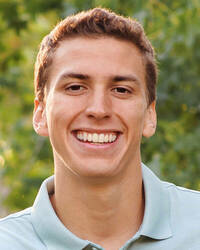
Faculty Advisor: Vlad Iluc, Department of Chemistry and Biochemistry
Isolation of Metallocyclobutane Intermediate for Ruthenium Catalyzed Olefin Metathesis as a Means for Bringing About More Sustainable Synthesis (Summer 2023)
Transition metal catalyzed olefin metathesis has been around since the 1950’s and is a versatile reaction important for a variety of synthetic processes academically and industrially. Although a variety of transition metal catalysts can be utilized, olefin metathesis is typically carried out with ruthenium carbene catalysts. Ruthenium is a rare transition metal that requires a significant amount of energy to extract and isolate. Furthermore, any residual ruthenium must be fully removed from pharmaceutical products that utilize ruthenium catalyzed olefin metathesis since ruthenium is harmful to the body and the environment. In recent years, a more sustainable and environmentally friendly catalyst alternative has begun to surface: iron. Iron is far more abundant and requires less energy to extract and isolate than ruthenium. It is also far safer to ingest and release into the environment. The Iluc laboratory has recently published an iron carbene that shows promising results in moving towards iron catalyzed olefin metathesis. Over the past few months I have successfully synthesized and characterized an analogous ruthenium carbene. This is significant because the iron system tends to involve NMR-silent compounds due to the paramagnetism exhibited by high spin iron carbenes. Thus, having an NMR-active analogous ruthenium carbene system could prove to be important for analyzing various reactions within the iron system.
With the Summer Slatt Fellowship research funding, I hope to contribute to the field of olefin metathesis by isolating and characterizing the first metathesis active ruthenium metallocyclobutane, a critical intermediate in understanding the mechanism behind olefin metathesis. Shedding light on the mechanism for this reaction is important because designing better catalysts requires one to understand all of the pieces of the mechanism. In this case, understanding the mechanism for the ruthenium system could lend valuable insight to the iron system and contribute to future work on iron catalyzed olefin metathesis. This could lead to the development of more metathesis active iron carbenes, which would bring about greater energy efficiency and reduce harmful waste production in synthetic processes that require olefin metathesis.
Konstantin Bauer
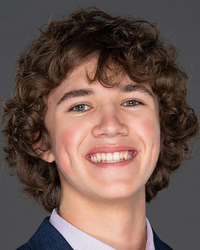
Combustion Synthesis and Characterization of Thorium Dioxide (Spring 2023)
Thorium dioxide (ThO2) is a promising fuel for next-generation nuclear reactors. This is due to the relatively high abundance of thorium, its higher efficiency, the fact that it produces less nuclear waste, and its better resistance to nuclear weapons proliferation. However, there are difficulties with using ThO2 in nuclear reactors related to the high sintering temperatures required to produce fuel pellets. Due to the potential that ThO2 holds for future nuclear reactors and its potential to aid in the search for the generation of clean, sustainable energy, it is vital to gain an in-depth characterization of thorium dioxide and thoroughly understand its properties.
This research project will explore the possibility of amorphous ThO2 preparation using a rapid and energy-efficient combustion synthesis method to decrease sintering temperatures. This project will have three main objectives. The first is to conduct a thermal analysis of reactive solutions producing ThO2, the second is to study the combustion synthesis of ThO2, and the third is to characterize prepared ThO2. By doing so, we can determine whether amorphous ThO2 can be produced by the combustion synthesis method.
Chloe Behringer
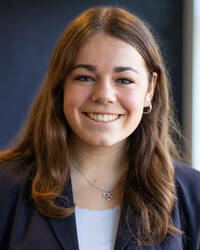
Faculty Advisor: Jonathan Whitmer, Department of Chemical and Biomolecular Engineering
Modeling Solvent Assisted Ion Transport in Structured Battery Electrolytes (Fall 2023)
Ionic liquid crystals are fascinating mesostructured materials which have the potential to be developed into future innovative battery technologies. The structure of these ionic liquid crystals, reminiscent of a lipid bilayer structure due to their ionic “heads” and long alkyl “tails,” enables a novel conduction mechanism mediated by connected charge domains within a solid glassy or gel-like domain formed by the organic tail groups. The difference in mobility between the two molecular species owing to this unique size and chemistry enables the possibility of creating single-ion conductors. Single-ion conductors serve as efficient transporters of only the necessary species for the needed device to function, resulting in increased charging rates and maximizing useful conductivity. Moreover, the tailgroups of these materials can be readily polymerized to reinforce the solid state, increasing the safety of lithium ion batteries by limiting dendrite formation, a common occurrence that can lead to short-circuiting of lithium-ion batteries with potentially explosive consequences.
This project is, in part, inspired by the alkyl-TSFI ionic liquid crystals synthesized by the Schaefer group here at the University of Notre Dame, which demonstrate single-ion conducting behavior within disordered layered aggregates formed in their solid state at room temperature. It remains an open question what the mechanism of charge transport is in these phases, and whether it is dominated by the connected ionic domains or by grain boundaries. Moreover, it is an open question to what extent the conductivity can be enhanced while maintaining single-ion conductivity. To answer these questions, we are building molecular dynamics models of the alkyl-TSFI ionic liquid crystals which we can use to explicitly interrogate their structure, mechanical response, and conductivity. To begin, the molecular simulation places these atoms into a layered structure, with polarized heads and ions forming a connected domain. Our first question is to explore the role of solvent molecules in fluidizing the ionic domain, and understand how much solvent may be added before disrupting the solid structure of the assembled liquid crystal molecules. Though solvent will create higher mobility in the ionic domain, tail groups will stay aggregated up to a point, lending the overall structure solid-like mechanical properties. Our next items will be to incorporate larger systems with a controlled density of grain boundaries to explore their influence on the conductivity of this system. Finally, we will explore the behavior these molecules exhibit upon polymerization, and use this to optimize liquid crystal topology and solvent content to obtain high conductivity in a single ion solid conductor. These molecular trends will not only be interesting to observe, but will also hopefully be broadly applicable in the development of long-lasting and effective energy storage devices.
Ryan Bencivengo
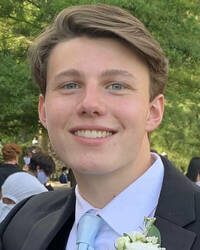
Faculty Advisor: Sergey Leonov, Department of Aerospace and Mechanical Engineering
Optimization of Energy Deposition Pattern at Plasma-Assisted Combustion (Spring 2023)
The general objective of the activity, where this research project is involved, is to study the transient physical and chemical processes associated with plasma-based ignition and flameholding in supersonic flow, which is related to prospective air-breathing hypersonic propulsion systems. This area of research and development is one of the US National Priorities and Defense Department's highest technical modernization priorities. The implementation of plasma-based ignition and flameholding would allow for fuel ignition and flame front stabilization in a supersonic duct without mechanical flameholders such as struts, wall-steps, or cavities. This could lead to a reduction in total pressure losses under non-optimal conditions, the shortening of a scramjet isolator and combustion chamber, enhancement in operation stability, and, as a result, the expansion of the capabilities in hypersonic vehicle operation. However, scientific and engineering research regarding plasma-assisted combustion is still in the fundamental phase. The Laboratory of Supersonic Combustion and Flow Control headed by Prof. Sergey Leonov is one of the world’s leaders in this area.
One very important task will be the proper optimization of the energy input for plasma-assisted combustion (energy deposition pattern). This task will require an understanding of the physical processes relevant to plasma-flow coupling and chemical kinetic processes associated with certain ignition phenomena. The power supply response function and the plasma generator geometry will control the energy deposition, and making it as efficient as possible is key to the success of this process. Specifically, the team is elaborating the hypothesis for a two-leveled plasma regulation system: high (10kV) voltage for the plasma initiation and a lower (2kV) voltage with a high (>10A) current for the plasma elongation and support. This goal is completely within the scope of the ND Energy activity.
The particular research tasks relevant to the Slatt fellowship Project, performed in collaboration with Prof. Leonov and graduate student Henri Doucet, will consist of the design and construction of the power supply and using this power supply for the supersonic combustion control test campaign. Specifically, it will consist of drafting a design of the high-voltage components arrangement within the power supply rack; assembling and testing of both the individual components and an entire assembly; adjusting the experimental set-up parameters to diminish secondary effects and excessive heating; providing parametric analysis of the testing data, including the waveforms of plasma initiation, and an initial electric field configuration; adjusting the waveforms by means of electrical circuitry when deviations from anticipated plasma behavior are found; report compilation and further planning.
Evan Bursch
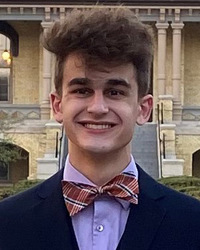
Faculty Advisor: Ryan McClarren, Department of Aerospace and Mechanical Engineering
Extending A Model of High-Order Implicit Shock Tracking For Inertial Confinement Nuclear Fusion (Spring/Fall 2023)
This past year, thanks to the generous support of the ND Energy Vincent P. Slatt Fellowship for Undergraduate Research in Energy Systems and Processes, I was able to begin developing a preliminary model of high-order implicit shock tracking for inertial confinement nuclear fusion. The basis of this research lies in the field of computational radiation hydrodynamics as applied to high-order implicit shock tracking. This model has the potential to aid the simulation of inertial confinement nuclear fusion experiments which directly accelerates the pursuit of net energy gain from nuclear fusion. The goal moving forward is to expand the scope of the model. Thus far, a limited one-dimensional space-time shock tracking simulation is operational, has been verified with standard benchmark tests, and was presented at an international conference. As the project continues, the objective is to extend into two and three-dimensional space-time problems and increase the accuracy of the radiation hydrodynamical model being used. These extensions would further validate the methodology and allow for more complex simulations of critical nuclear fusion reactions that are too computationally costly or downright impossible using traditional methods. By helping advance the field of nuclear fusion research I hope to do my part in battling the detrimental effects humanity is having on the environment and hasten the much-needed transition away from fossil fuels. Nuclear fusion holds the key to a global future of clean, safe, and low-cost energy for all.
Sam Chen
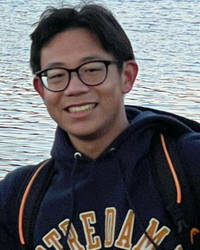
Polymers in Next-generation Rechargeable Batteries (Summer 2023)
Due to environmental concerns and limitations of fossil fuel resources, society is shifting towards renewably generated electricity, resulting in a higher demand for high-quality batteries. However, commercially used electrolytes, an essential part of the battery, are highly volatile and flammable. As future engineers, it's our duty to develop batteries with higher energy capacity for market demands while improving their safety and reusability, therefore I chose this project.
My research focuses on investigating the impact of polymers in commercial battery electrolytes. Safety tests suggest certain polymers can reduce the heat generated during batters' catastrophic failure. In other words, polymers help improve Li-ion batteries' thermal stability. However, they also act as boundaries when electrons exchange between two electrodes and decrease battery conductivity. It's important to find a balance between the two concerns, safety and performance. In the past year, I've been working on data analysis of electrolytes with PFPEDA (perfluoropolyether diacrylate). The results suggest that this polymer has both good conductivity and potential stability. The main objective of my research currently is to finish collecting the transference number of PFPEDA electrolytes, show data reproducibility, and investigate battery recharge-ability. However, I'm sure that some goals will be accomplished by the end of Spring 2023 with new goals being set.
Elliot Como
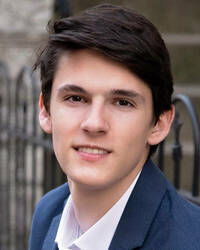
Faculty Advisor: Ruilan Guo, Department of Chemical and Biomolecular Engineering
Architecting Self Semi-interpenetrating Network (ss-IPN) Structure in PIM-1 Membranes for Enhanced Physical Aging Resistance (Spring/Fall 2023)
Polymer-based membranes for gas separations represent a great opportunity for reducing cost, energy usage, and CO2 emissions in chemical separation processes. One of the primary concerns with using polymers for gas separation is physical aging. Aging occurs due to polymer chain relaxation to approach thermodynamic equilibrium of chain packing. Aged polymers have lower fractional free volume (FFV) and are more densely packed which corresponds to a permeability drop. The PIM-1 polymer is a representative high-free-volume glassy polymer membrane material, showing ultrahigh permeability and good selectivity. However, PIM-1 is viewed as imperfect for industry applications due to its rapid physical aging. Existing preventative methods for physical aging, such as random crosslinking, also cause large permeability losses. This research attempts to address this issue by exploring a macromolecule design of self-semi-interpenetrating networks (ss-IPN) where linear PIM-1 chains penetrate through cross linked unimodal networks of PIM-1 to mitigate crosslinking-induced permeability loss and prevent the free volume collapse via the rigid network 'scaffold'. The structure property relationship will be investigated by varying the membrane composition through the linear PIM to oligomer PIM ratio and the crosslink density through the use of different oligomer lengths. The goal is to understand how this crosslink system effects membrane aging over time in regards to permeation performance and structural properties such as FFV and d-spacing.
Lauren Farrell
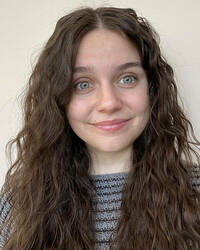
Gaining Access to the Reaction of the Reduction of Carbon Dioxide to a Usable Fuel (Summer 2023)
One of the major contributions to global warming is the release of carbon dioxide into the atmosphere from the burning of fuels. If the carbon dioxide product from the burning of a fuel could be converted back into that fuel, this would allow for the process to have a net zero emission of carbon dioxide. However, in order to produce an ideal fuel from carbon dioxide—like methanol—it would need to be reduced by six electrons. This is difficult because it is hard to reduce carbon dioxide by more than two electrons. This research project aims to discover a new system for the selective reduction of carbon dioxide to a usable fuel. If attainable and energy-efficient, this would allow for the fuel to become a carbon-neutral carrier of energy and address the problem of carbon dioxide warming up the atmosphere.
The objectives of this research project include further exploring redox reactions between a nucleophilic iridium complex and electrophilic carbon dioxide. It is hoped that by using this iridium complex to hold the carbon dioxide, the proton and electron flow needed to do multi-electron reductions without releasing the carbon until it’s at the appropriate oxidation state can be achieved. The research project will be focused on preparing and studying the iridium complex combined with carbon dioxide at different oxidation states, determining how to interconvert between them, and studying the selectivity and efficacy of these reactions. This will allow us to see and further study the proposed mechanisms for reducing the carbon dioxide enough to be able to have more useful products.
Gabe Goertz
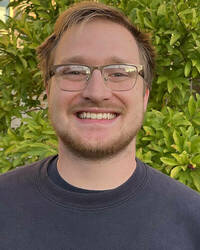
Nanocrystal Surface Electrostatic Effects on Tethered Catalyst Potentials (Summer 2023)
Colloidal semiconductor nanomaterials introduce a new layer of customizability to the field of catalysis. By inducing electric fields, these semiconductor nanomaterials are able to alter the electronic landscape of bound ligands—in the case of a bound catalytically active species, this introduces the possibility of adjustable reactivity and rate parameters.
My research, specifically, has been focused on the synthesis and development of carboxylate-functionalized ligands centrally bound to a transition metal (currently Ni) and peripherally bound through carboxylate groups to a CdSe nanoparticle. In the future, these CdSe nanocrystals with bound Ni complexes will be electrochemically characterized, then tunability and electrochemical response will be investigated through variation in nanomaterial structure and shape.
Eva Homberger
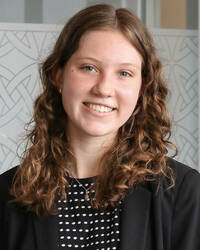
Faculty Advisor: Peter C. Burns, Department of Civil and Environmental Engineering and Earth Sciences
Batch Sorption of U60 Nanocluster to Muscovite in the Presence of Electrolytes (Spring 2023)
This project will build upon the line of research studying the behavior of uranyl peroxide nanocluster U60 in the presence of minerals. Specifically, the immediate goal of this project is to assess the impact of various conditions (cation, pH, concentration) on the sorption of U60 to muscovite. In the long-term, once a suitable system is identified, in-situ experiments of the sorption process could potentially be performed using atomic force microscopy (AFM) and time-resolved laser-induced fluorescence spectroscopy (TRLFS) in order to determine the sorption mechanisms. The motivation for this project stems from the looming issue of the Hanford Site in Washington State, a decommissioned nuclear production complex that now has become a massive national liability due to the scale of hazardous waste and contamination present at the site. Waste at Hanford is stored in tanks, multiple of which are leaking. The potential for uranium mobility in the environment poses a threat to safety, particularly if the waste reaches groundwater. Furthermore, evidence suggests that clusters, such as U60, are forming in the waste slurry. As a result, it is critical to acquire a deeper understanding of the behavior of U60, especially in regards to sorption as a main factor in slowing radionuclide mobility in the environment. Nuclear energy presents a carbon-free and scalable solution to the looming energy crisis, but proper waste treatment is necessary to ensure that nuclear energy is sustainable in the long-term.
I will conduct three main sets of experiments: isotherm experiments, kinetic experiments, and control experiments. The isotherm experiments will help determine the sorption capacity, or the amount of U60 expected to sorb onto muscovite depending on the electrolyte and pH of the system. Next, the kinetic experiments will reveal the sorption curve of the systems; this will show how much U60 is sorbed onto muscovite as a function of time. Finally, the control experiments will confirm that the electrolyte and pH are indeed the independent variables influencing the sorption. In the end, I aim to understand how different electrolytes and pH levels impact the sorption of U60 onto muscovite, and propose plausible theories for the sorption mechanism.
Thomas King
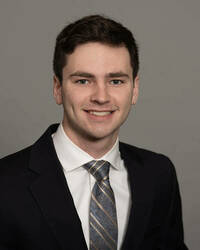
Faculty Advisor: Ryan McClarren, Department of Aerospace and Mechanical Engineering
Developing Metadata for Nuclear Fusion Models (Fall 2023)
Machine learning is becoming an integral part of technology development. With fusion energy research, the use of machine learning tools is hampered by the lack of publicly available data that adhere to the FAIR data principles (https://www.go-fair.org/fair-principles/). This work in collaboration with Prof. McClarren, an affiliate of ND Energy, and the International Atomic Energy Agency (IAEA) is to build an example database of simulations of inertial confinement fusion experiments.
This work will take existing methods and capabilities developed at ND and increase their global impact by making the results more visible and to enable others to benefit from that work. We will be executing a series of simulations and store the output data in machine readable form with standardized metadata. This data will be shared with other international groups. We will work with these other groups to confirm that our standards for description of the data are sufficient.
This work will involve four phases: 1) determining the scope of the data set to be produced, 2) producing the data, and 3) developing our metadata description, 4) developing a machine learning use case. Part one of the project will involve becoming familiar with the simulation codes and working with Dr. McClarren and his graduate students to decide what simulations we need to complete to populate the data set. These simulations will be executed during phase 2. Simultaneously with the data being produced we will develop the metadata descriptive tools. This work will involve determining the format of the metadata and other requirements. Finally, once the data has been produced, a machine learning use case will be developed to use a tutorial for future users of the data.
Lauren Martin
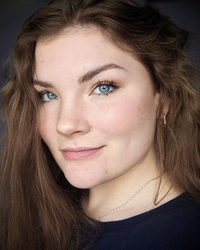
Faculty Advisor: Prashant Kamat, Department of Chemistry and Biochemistry
Energy Transfer in Lead Halide Perovskite-Molecular Hybrid Assemblies for Light Harvesting Applications (Summer 2023)
As consumption of energy increases in our growing world, we find ourselves in need of renewable and sustainable sources. One such solution, solar energy, is an underutilized and abundant resource with a promising future. However, current solar energy devices lack the energy capture and conversion efficiency necessary for widespread implementation. Thus, we turn for inspiration to the photochemical architecture that nature has perfected over millions of years - plants. In photosynthesis, plants harvest photons by absorbing them through pigments, and then transferring them down a photochemical cascade through which the light is then converted to energy. In recent years, significant research effort has been expended on funneling energy from semiconductor nanocrystals to sensitizing dyes by designing hybrid assemblies that can capture photons over a wide range of visible and near-infrared region. A significant advancement in the field is tailoring such assemblies to initiate nonlinear optoelectronic processes like triplet-triplet annihilation (TTA) and singlet fission (SF) which theoretically overcome the limitation of power conversion efficiency in conventional photovoltaic devices. Here, we focus on the use of lead halide perovskites (CsPbX3, X = Br, I) as the sensitizer, and rubrene and perylene derivative (DBP) as the sensitizing molecules. Lead-halide perovskites are materials that show promising trends for future semiconductor research and applications, which now have power conversion efficiencies similar to the best silicon solar cells.
We investigated how the systems interact at rubrene-DBP, CsPbBr3-rubrene, and CsPbBr3-DBP interface by preparing the corresponding films via spin coating. We established singlet energy transfer from rubrene to DBP at rubrene-DBP interface. We employed absorption, emission, photoluminescence decay and excitation spectra to confirm the singlet energy transfer. Time-resolved absorption and emission measurements helped to measure the rate constant and efficiency of energy transfer. Preliminary studies on excited-state interactions show singlet energy transfer at CsPbBr3-rubrene, and CsPbBr3-DBP interface. Altogether in CsPbBr3-rubrene-DBP mixed film, we observed cascading singlet energy transfer from CsPbBr3 to rubrene and then to DBP. Further studies must be done to obtain the efficiency and rate of the energy transfer. The bandgap energy of the CsPbBr3 nanocrystals can be reduced by alloying it with iodide. As we halide alloy bromide to iodide in CsPbX3 nanocrystals, the band gap energy decreases, and the emission redshifts. By iodide alloying, we can suppress the singlet energy transfer and facilitate the triplet sensitization of rubrene. We will employ iodide alloying in future studies to investigate the sensitization of rubrene by CsPb(BrxI(1-x))3 nanocrystals.Taken together, this project highlights the potential that perovskite nanocrystals have as a sensitizer for applications in photovoltaics and photocatalysis.
John Moore
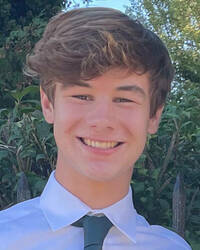
Faculty Advisor: William Phillip, Department of Chemical and Biomolecular Engineering
Development of Novel Nanofiltration Membrane Functionalizations for the Specific Ion Rejection of Cobalt and Lithium (Summer 2023)
Membrane-based nanofiltration (NF) technology has come to the forefront of research on water purification and filtration due to its energy efficiency. For example, NF membranes have been used to recover trace amounts of heavy metals from wastewater. Membranes separate mixtures by modulating the relative flux of different chemical species. There are multiple ways to achieve this relative flux. Membranes can inhibit the transfer of the target solute, leading to the rejection and purification of the resultant solution. In this way, membranes can be used to separate solutions ideally by achieving 100% rejection of one species while another species would not be rejected. In this project, the target species are metal cations lithium and cobalt, which are important ions for renewable energy storage and production.
Research on specific functionalization is ongoing, with researchers exploring modifications to the pore wall chemistry with both positive and negative charges along the membrane surface to inhibit target solute transfer. The principles of research on pore size manipulation are detailed in the paper entitled Microstructure manipulation in PVDF/styrene-maleic anhydride copolymer composite membranes: Effects of miscibility on the phase separation (Jour. Sep. and Pur. Tech. 2021, 263, 118371). In addition, research on charge manipulation along the membrane surface is detailed in the paper entitled Interfacial Junctions Control Electrolyte Transport through Charge-Patterned Membranes (ACS Nano 2019, 13, 7, 7655–7664). I have experience fabricating membranes and functionalizing them with secondary amines that carry a positive charge at neutral pH. This modification of the pore wall has been shown by lab members to reject positively charged ions from solution. Similar trends in rejection are observed for negatively charged membranes as well.
However, these specific functionalization protocols haven’t been integrated with novel inkjet printing technology, which allows for reproducible and accurate chemical patterning of the membrane surface. Inkjet printing has a proof of concept, detailed in a paper titled Template Synthesis of Nanostructured Polymeric Membranes by Inkjet Printing (ACS Appl. Mater. Interfaces 2016, 8, 5, 3386–3395). We still don’t fully understand how patterning membranes with positive or negative charges affects the transport of charged species. It’s interesting because due to the phenomena that occur concerning these charged membranes, it may be possible to speed up the transfer rate of one ion while keeping the other the same or even slowing it down.
This project will explore the inhibition of the mass transfer of heavy metal ions like lithium and cobalt, across the membrane surface by exploiting their positive charge. Functionalization will occur after casting, through a treatment of the membrane to chemically alter its surface. This project will work towards attaching positive and negatively charged moieties within the membrane structure to inhibit the transfer of heavy metal ions across the membrane surface. The importance of a project that aims to mitigate the effects of “e-waste” primarily from electronics carrying important materials, is detailed in the paper Optimal Diafiltration Membrane Cascades Enable Green Recycling of Spent Lithium-Ion Batteries (ACS Sustainable Chem. Eng. 2022, 10, 37, 12207–12225).
Specific Aim 1: Develop a reproducible protocol for functionalizing large membrane sheets.Protocols for the fabrication and functionalization of membrane sheets will be developed to couple azide moieties with alkynes. Azide and alkyne reactions are familiar to the lab and well documented in literature. There will be a positive moiety, propargylamine, and a negative moiety, in the form of an alkyne sulfonate group. My previous efforts within the lab have yielded membranes with consistent permeabilities for a wide range of solvents. Due to this experience, my efforts will be geared toward functionalizing these membranes. Nuclear magnetic resonance (NMR) and Fourier Transform Infrared (FTIR) spectroscopy will be used to analyze the chemical composition before and after functionalization. With respect to FTIR, the parent membrane possesses a distinct azide peak at 2100 cm-1 that will disappear when the azide is consumed by the functionalization protocol. Similarly, the hydrogen atoms within the polymer have specific NMR peaks that will shift when the polymer is functionalized. Additionally, I expect new NMR peaks, that correspond to the hydrogens of the added moiety, to appear.
Specific Aim 2: Quantify the impact of membrane functionalization on target solute transport.To separate lithium (Li) and cobalt (Co), I will conduct filtration experiments on nonfunctionalized (control) and functionalized membranes. Under neutral pH conditions, the amine that will be introduced to the membrane is protonated and possess a positive charge. Positively charged heavy metal ions will be repelled (i.e., rejected) due to electrostatic forces. Although both Li and Co are charged, I hypothesize that they will be rejected at different rates because of the charge differential between the two ions (cobalt has a 2+ charge and lithium only has a +1 charge). Additionally, lithium is significantly smaller than cobalt in terms of hydrated radius.
The heavy metals will be dissolved in water, and the resulting solution added to a membrane testing apparatus. This solution will then be pushed through the membrane under ≈ 60 psi. of pressure. The solution that has permeated through the membrane is collected in a vial. Both the receiving and feed solutions will be analyzed for the contents of each ion. After the test is run, the resultant solution will be analyzed using inductively coupled plasma optical spectroscopy (ICP - OES) to detect metal cations (Li+ or Co2+) in the aqueous solution. To see if the presence of multiple ions in solution alters membrane performance, the mixed-ion experiments will be conducted analogously. The concentrations of each ion in solution will be altered to examine if the membrane performance remains the same in non-equimolar solutions.
Specific Aim 3: Develop protocols for executing the functionalization using inkjet printing technology.Inkjet printing technology enables the functionalization of membranes with multiple functionalities, to create chemical patterns on the membrane surface and improve functionalization consistency. Inkjet printing technology will be incorporated similarly to the protocol developed in the paper Template Synthesis of Nanostructured Polymeric Membranes by Inkjet Printing (ACS Appl. Mater. Interfaces 2016, 8, 5, 3386–3395). The solutions used to functionalize the membranes will be used as “inks” to print the functionalizations in a patterned way on the membrane surface. The positive and negative functionalizations will be printed on the membrane surface simultaneously, and both the surface area and the geometry of the printed inks will be varied to test for mixed ion rejection. I hypothesize that there will be a hexagonally arranged geometry of species-specific functionalization that will allow for Lithium to be transferred faster than Cobalt, which will allow for more selective separations.
Zachary W. Muetzel
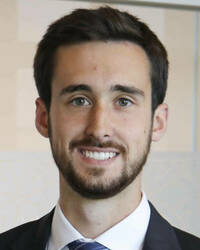
Faculty Advisor: William Phillip, Department of Chemical and Biomolecular Engineering
Development of 3D-printed Modules to Evaluate the Performance of Photoresponsive Polymer Membranes (Spring 2023)
“Smart” materials have gained traction in recent years due to their ability to respond selectively to stimuli in a variety of applications and industries. In separation processes, smart membranes are being examined for their ability to change pore size when stimulated. This increased control can be leveraged to modulate mass transfer across the membrane surface, thereby increasing the flexibility and versatility of the material. Photoresponsive membranes, those that undergo chemical changes when exposed to light of a specific wavelength and intensity, are of particular interest as light is a cheap, safe, and chemical-free stimulus that can alter membrane configuration.
Photoresponsive membranes have already been synthesized, such as those detailed in the paper entitled Smart light responsive polypropylene membrane switching reversibly between hydrophobicity and hydrophilicity for oily water separation (Jour. Membr. Sci. 2021, 638, 119704). However, no dead end stirred cell (the accepted membrane testing configuration) designs currently exist that incorporate light sources to evaluate their performance. Such performance metrics include mass transfer across the membrane as a function of light intensity or angle relative to the membrane and the minimum ratio of lit to dark time the membrane needs to achieve a desired level of separation.
The objective of the proposed research is to create a modified stirred cell that includes light sources positioned to provide consistent, direct light to the active surface of the smart membranes in situ. Multiple configurations will be created to probe the effect of changing light position relative to the membrane surface. My previous work creating an automated membrane testing apparatus, detailed in the paper entitled Device for the Acquisition of Dynamic Data Enables Rapid Characterization of Polymer Membranes (ACS Appl. Polym. Mater. 2022, 4, 5, 3438–3447), provided opportunities to design custom 3D-printed parts, integrate those components with established membrane testing protocols, and automate the protocols to execute with minimal experimenter interaction. This prior experience prepares me for the proposed research objective. Eventually, the modified stirred cell that I will develop could be incorporated into the ADD environment. This would allow for automation of testing protocols unique to photoresponsive membranes, such as switching the light sources on and off automatically over the course of an experiment and observing the corresponding changes in membrane throughput. This would provide insight into the property change kinetics of photoresponsive membranes as a result of stimulus state changes.
Omar G. Muñoz
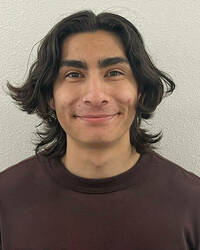
Faculty Advisor: Yahya C. Kurama, Department of Civil and Environmental Engineering and Earth Sciences
Reinforced Concrete Designs for Large-scale Nuclear Building Structures (Summer 2023)
This project focuses on developing accelerated construction methods to improve building efficiencies and significantly lower the costs of building large-scale modularized reinforced concrete components for nuclear power plant structures. Muñoz aims to develop computer-based visualizations and laboratory prototyping of the construction methods investigated by the project.
Daniel O'Connor
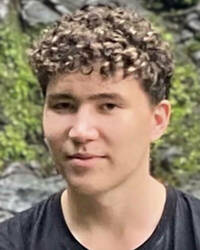
Faculty Advisor: Casey O'Brien, Department of Chemical and Biomolecular Engineering
Integrated CO2 Capture and Conversion (Summer 2023)
Current sorbent-based CO2 capture processes are prohibitively expensive due to their multistep and energy intensive nature. The need to compress, transport, and store CO2 also renders these processes inefficient. This project looks to integrate CO2 capture and conversion into a single unit process through the use of catalytic membranes. To do this membranes must be able to fulfill certain criteria: The membrane must, separate CO2 from air; catalyze CO2 conversion on the permeate side of the membrane with high activity and selectivity; couple and match selective CO2 uptake and transport rates across the membrane with CO2 conversion rates on the opposing side of membrane; and be stable for extended operation while operating at mild temperature (<100 C) and pressure (1 atm). The envisioned membrane is polymer based membrane with amine groups attached to the polymer chain to capture and facilitate the transport of CO2. The membrane would also include catalytic groups to catalyze conversion of CO2 on the permeate side of the membrane. It has been found that polyvinyl pyridine based (P4VP) membranes show promise in the capture and conversion of CO2. This compound can be modified through quaternization using alkyl halides as well as by metalation with various salts or metal nanoparticles. These modifications can help to enhance its catalytic behavior and carry out complex transformations. P4VP-based polymers provide a highly tunable platform for demonstrating the potential of amine functionalized membranes to integrate CO2 capture and conversion to a variety of fuels and chemicals. The main objectives of my research will to clarify how P4VP- based molecules catalyze conversion of CO2 to cyclic carbonates and how operating conditions influence this conversion as well as how the integrated CO2 transport and conversion process works under different conditions. My research focuses on the catalysis portion of this project.
Delaney Ryan
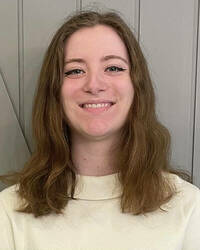
Faculty Advisors: Ian Carmichael, Aliaksandra Lisouskaya, Notre Dame Radiation Laboratory
Radiation Stability of Organics in the Nuclear Waste Extraction (Summer 2023)
This semester, I have been fortunate enough to be in the inaugural class of Sustainable America, a cross-disciplinary class that focuses on learning how we can use Catholic Social Teachings, American Studies, and our major (Chemical Engineering) to create a more sustainable world. As an engineer interested in nuclear energy, my professor has pushed me to focus on the environmental and energy components of the class, and more specifically, the potential for nuclear energy to become sustainable. Research, especially the kind of research that can be done through programs like the Slatt Summer Fellowship, is essential so scientists can help create a more sustainable tomorrow. I believe that with topics like nuclear energy, there are certain stigmas and concerns attached to it (like the events that took place at Chernobyl, Three Mile Island, and Fukushima Daiichi) that make people afraid to trust the good that can come from its wide-scale implementation. The work we do in the Lisouskaya Lab has the potential to revolutionize nuclear energy because we are looking into better ways to safely recycle spent nuclear fuel and harness the remaining high-level for further use. The more research we do and studies we publish in our effort toward sustainable nuclear energy, the less stigma may be attached to the subject. Our findings may help to increase safety to preclude future incidents resulting in more widespread use and a more sustainable future.
As a chemical engineer, I’m allowed to add a concentration in my senior year. I plan on adding an energy concentration to further my studies in sustainable energy sources like nuclear energy. Over Christmas Break, I discovered Dr. Aliaksandra Lisouskaya was conducting research on campus and I contacted her to see if I could learn more about her work. Since January, I have been working with Dr. Lisouskaya’s postdoctoral student Iliya Sosulin twice a week on various experiments involving EPR and the linear accelerator (LINAC) machine. In our preliminary experiments, we used the spin-trapping EPR method to characterize the radiolytic stability of organic ligands using tributyl phosphate (TBP), which is a component of the most widely used solvent extraction PUREX (Plutonium - URanium EXtraction) process. The radiolytic stability of TBP was studied using a spin trap α-(4-Pyridyl-1-oxide)-N-tert-butylnitrone (POBN). The spin trapped EPR method allows us to identify short-lived radicals as spin trap adducts, which may be characterized by a specific splitting spectrum for each adduct. An important advantage of the spin-trapping method is that stable adducts have well-resolved spectra and can be analyzed over time after irradiations at room temperature. Total trapped radical yields were measured as a function of POBN concentration and as a function of radiation dose. Our plan is to study a new system with other organic ligands, organophosphorus compounds, and diamides. We plan to use the rapid-flow EPR to examine other radicals like the Ti3+/H2O2-Fenton system, which generates inorganic (HO●) and organic (R●) radicals in the EPR cavity. Because of the way the EPR machine was designed, we can detect in-situ radicals in solution if we rapidly mix the reagent streams before putting them in the EPR. EPR is essential to help us determine the kinetics of radical reactions. We will need to use quantum chemical calculations to determine the identities of the radicals derived in our experiments. The results of our work this summer will be important in determining the radiation stability of organic ligands, especially since they have been proposed as potential extractants of spent nuclear fuel.
Bennett Schmitt
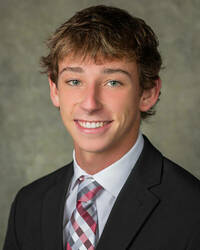
Faculty Advisor: Antonio Simonetti, Department of Civil and Environmental Engineering and Earth Sciences
Investigating Rare Earth Element Mineralization Within Fenite Alteration Zones (Summer 2023)
Rare Earth Elements (REEs) play a critical role in the expansion of clean energy technology. These elements form key components of batteries for electric vehicles and other energy storage devices, but their low concentration throughout Earth’s crust requires inefficient, energy-intensive extraction processes. In the last few decades, carbonatites—a carbonate-rich subset of igneous rocks—have been shown to harbor the most abundant REE deposits in the world. Carbonatite complexes have been studied extensively, but little attention has been given to the nearby rocks that are chemically altered by the intrusion of carbonatite magma. These rocks, known as fenites, show a similar REE enrichment and represent an untapped reservoir of key elements and minerals that can help to accelerate the transition to a clean energy future.
This project seeks to investigate the mechanisms responsible for the concentration of REEs in fenite alteration zones. By juxtaposing mineralogical, chemical, and isotopic investigations of fenites and their associated carbonatites, this project will provide novel insight into the geochemical processes controlling REE movement and enrichment in these complexes. Samples to be studied have been gathered from three of the largest carbonatite-associated REE deposits in the world, and preliminary analyses have confirmed the suspected profound changes in the fenites relative to unchanged nearby rock. This project stands to provide a heightened understanding of the elemental nature of these largely unexplored alteration zones, elucidate a path for more sustainable and efficient production of clean energy technologies, and advance energy storage capabilities.
I will be responsible for bulk and trace element analyses, as well as isotopic investigations of the carbonatite and fenite samples. Through the use of electron microprobe analysis, laser ablation, and mass spectrometry, I will work to understand the similarities and differences between the carbonatites and their associated fenites. Through elemental analysis, I will be able to identify patterns of variations in chemical composition of these samples. Isotopic studies will allow me to investigate the genesis of the carbonatites and examine how they modulate such a vast presence of REEs. By parsing out these chemical and isotopic relationships, I can begin deducing the processes leading to REE enrichment in fenite alteration zones and gain an understanding of how these deposits may be targeted and efficiently extracted to supply a growing need for energy storage technologies.
Rubén E. Torres
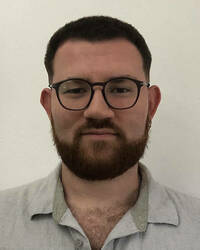
Faculty Advisor: Patrick Fay, Department of Electrical Engineering
Simulation and Characterization of Wide Band Gap Power Devices (Summer 2023)
This research project focuses on the experimental characterization of high-power GaN-based devices and the numerical simulation of devices, intended for future-generation high-efficiency power conversion systems.
Evan Wood
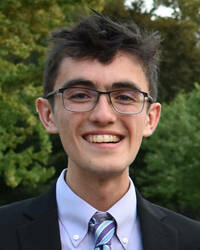
Faculty Advisor: William Phillip, Department of Chemical and Biomolecular Engineering
Selective Ion Transport with Imidazole Membrane Ligands (Summer 2023)
Of the different forms of separation processes, on-going research in membrane separation processes has the potential for significant gains in the energy efficiency and environmental cost of specialized separation. For example, the increasing prevalence of Lithium-Ion batteries, the potential environmental hazard these spent batteries pose to the environment, and the high material cost of Cobalt within these batteries, Li-Ion battery recycling processes must be further developed. A critical step in the recycling of Lithium-Ion batteries involves the acid leaching of metal ions from the electrode resulting in a product stream of Cobalt, Copper, Nickel, Manganese, and Lithium ions dissolved in water in the presence of acid. Currently, liquid-solvent extraction and salt precipitation processes are used to separate and recover the target metals; however, significant drawbacks of these processes include the generation of a large volume of potentially environmentally-hazardous waste streams, or the evolution of toxic gasses and complicated separation steps because of the presence of competing ions in solution. A possible alternative to these harsh separation methods is membrane separation.
Typical membrane separation processes face significant hurdles in separating similarly sized, and similarly charged molecules. One way to overcome this limitation is to add ligands that can increase the thermodynamic partitioning of ions from the solution, which subsequently diffuse through the membrane pores. Different ligands are being explored due to the greater control and tunability of the attractive interactions between the ligand and target ion. The goal is to achieve an intermediate ligand transient interaction that does not either attract target molecules too greatly, such that membrane transport is hindered significantly; or, does not act too weakly such that transport properties would not be meaningfully affected. This research will focus on establishing structure-function relationships for electron-rich ligands which can mediate the transport of ions by forming ligand-metal complexes. Potential applications of this technology could be separating Copper, Cobalt and other metal ions in recycling streams produced from the acid leaching from Lithium-ion batteries.
This research project will focus on synthesizing GMA-copolymer membranes with an imidazole functional group, and an indole group: it is believed these ringed organic groups have interesting transient interactions with positively charged ions. The extent to which the reaction pathway added the desired ligand to the membrane will be investigated. Then, the permeability characteristics of the membrane will be determined. Crucially, the transport effects the ligands may have on the diffusion of Cobalt and Copper across the membrane, will be investigated; especially as the phenomena is affected by the presence of multiple components of varying concentration in the feed stream.
Evan also received First Place in the inaugural Summer 3MT Competition for his presentation on "Better Recycling for Lithium-Ion Batteries".